Two previous articles were desk studies of the the ARRL EFHW kit transformer, apparently made by hfkits.com:
This article documents a build and bench measurement of the component transformer’s performance, but keep in mind that the end objective is an antenna SYSTEM and this is but a component of the system, a first step in understanding the system, particularly losses.
The prototype
Albert, KK7XO, purchased one of these kits from ARRL about 2021, and not satisfied with its performance, set about making some bench measurement of the transformer component.
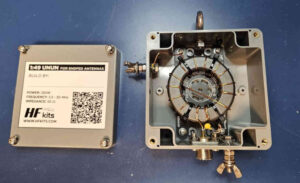
Above is Albert’s build of the transformer.
The kit parts list is as follows:
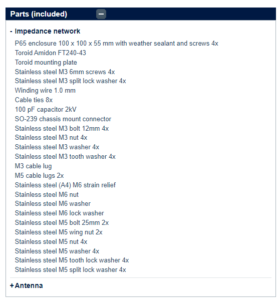
Magnetics
The first point to note is that Amidon’s 43 product of recent years has published characteristics that are a copy of National Magnetics Group H material (though they changed the letterhead).

Above is a side by side comparison of the NMG H material datasheet and Amidon 43, the Amidon appears to be a Photoshop treatment of the NMG.
Fair-rite have been a long term manufacturer of a material they designate 43 (which has changed over time), and it has been resold as such by many sellers. NMG H material is somewhat similar, but to imply it is equivalent to Fair-rite 43 might be a reach.
Overall design
I might note at the offset that the design is not original, there are countless articles on the net describing a 2:14 turn on a ‘FT240-43’ transformer for an EFHW using exactly the same winding layout. As discussed in other articles on this site, 2t is insufficient for operation down to 3.5MHz using Fair-rite #43, even worse for the National Magnetics H material published as Amidon 43.
Measurement
Measurements were made with a NanoVNA-H4. It is not a laboratory grade instrument, but well capable of qualifying this design and build.

Albert performed a two port measurement using the setup above. This places a nominal load on the transformer, and the voltage division of the series resistor and Port 2 input impedance is used to correct the measured s21 figure.
So let’s take the measurements and calibrate a SimNEC model of the transformer.

Above is the model using Fair-rite #43 material calibrated to measured leakage inductance and InsertionVSWR. The reconciliation of model (magenta) with measurement (green) is good. The equivalent Fair-rite part is 5943003801.
Let’s compare measurement to the same model but with Amidon 43 material (NMG-H):

Reconciliation is very poor at lower frequencies, the measured transformer appears to have significantly higher permeability.
Measure core complex permeability
Let’s take a diversion for a moment and put this question to bed.

Above is complex permeability calculated from measurement of a 1t winding on the core used above compared with the Fair-rite #43 2020 published data. Keep in mind that tolerances on ferrite are relatively wide, these two reconcile very very well, there is no doubt in my mind that the material is Fair-rite #43. This questions the seller’s specified parts list.
A similar plot against NMG-H shows a stark difference.
InsertionVSWR and ReturnLoss
So let’s return to the saved .s2p file from the two port measurement.

Above is a plot of bench measurement of ReturnLoss and (related) InsertionVSWR for the transformer with a nominal load.
The InsertionVSWR might look acceptable, but using InsertionVSWR as a single metric is a very limited view.

Above is a plot of InsertionLoss (-|s21|dB), and its components MismatchLoss and (Transmission) Loss. See Measurement of various loss quantities with a VNA for discussion of Loss terms.
Let’s dismiss performance below 7MHz, the plot shows it has insufficient turns.
Importantly, (Transmission) Loss is around 1dB at 7MHz, so 20% of the input power is converted to heat in the core and winding, mostly in the core. If you look back to the first SimNEC screenshot, the model predicts just under 1dB Loss, so measurement reconciles well with the prediction model.
Experience and measurement of Loss and thermographs informs that a transformer of this type in this type of enclosure is not capable of more than about 10W of continuous dissipation in typical deployments, less if it is in direct sun in a hot climate. That means the transformer is not likely to withstand more than about 50W average input power without damage or performance degradation.
Now that might be quite acceptable to some users, gauging by the number of web articles and Youtube videos recommending this, a lot of users apparently.
Credit
Credit to Albert for his interest in understanding these things, careful measurement of the prototype, and preparedness to dismantle the prototype for science.
Summary
So let’s end this article with the results of desk study and measurement:
- though the kit specifications state the core is Amidon #43, the sample kit is almost certain to contain a Fair-rite 43 core which is significantly different;
- two port measurement of the sample of one with nominal load showed around 1dB of Loss at 7MHz;
- MismatchLoss grows rapidly as frequency is reduced below 7MHz suggesting it has insufficient magnetising impedance, a result of insufficient turns;
- the winding configuration is not optimised for leakage inductance;
- popularity is not a good indicator of performance.
Where to from here?
These problems beg a redesign and measure of the transformer… more to follow.
Last update: 7th September, 2024, 6:19 AM